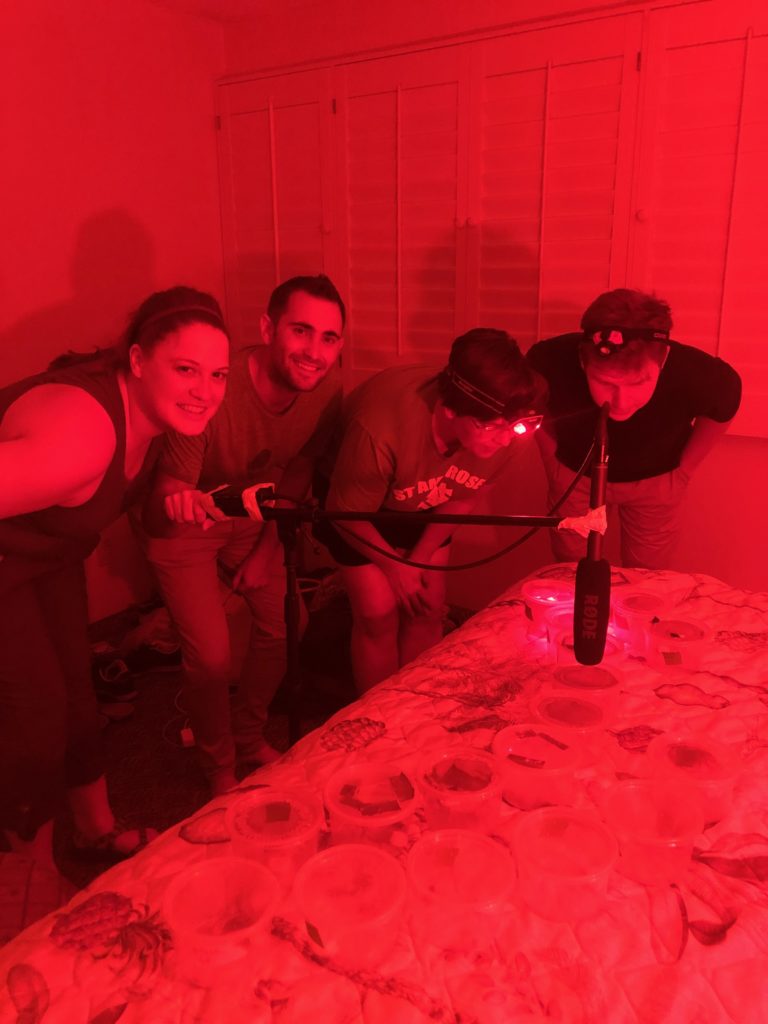
The activities are as follows:
- Teacher Guide
- Student activity, Graph Type A, Level 3
- Student activity, Graph Type B, Level 3
- Student activity, Graph Type C, Level 3
- Scientist Profile on Project Biodiversify
- Digital Data Nugget on DataClassroom, Part 1
- Digital Data Nugget on DataClassroom, Part 2
- Grading Rubric
Animals use many types of mating signals to attract mates. Some of these signals are probably familiar to you, like the bright colors of birds’ feathers, complex courtship dances of fish, and loud calls of frogs. In crickets, males rub their wings together to produce chirping mating songs that attract females. However, in one species of cricket, these mating songs have led to an issue – while they attract females towards the male, they also attract parasitoid flies. These flies kill the crickets by eating them from the inside out! Parasitoids are animals that lay their eggs in another organism’s body. The eggs develop and usually kill the host.
About twenty years ago, scientists discovered male Pacific field crickets in several spots in Hawaii had stopped making songs. By looking at their wings and DNA, scientists were able to find the exact genetic mutation causing their silence. This change in DNA made some crickets to grow with flat wings that made no sound. Males with this mutation are able to escape detection by the parasitoid flies. However, being silent also posed a struggle because flat winged males could no longer use songs to attract female mates. Scientists waited and watched – would a new way to attract females evolve over time, one that is audible to females, but not to the flies?
Robin is a scientist who has been studying the mating signals in these crickets for many years. One summer, Robin was working in Hawaii and brought a Tupperware container full of crickets into her room. Suddenly, she heard what sounded like a purring cat, but there was no cat in sight. She soon realized the sound was coming from her container of crickets. This song was unlike anything ever observed before in crickets.
Robin thought that this purring song might be the beginning of the evolution of a novel signal that could be detected by female crickets. If purring is a mating signal, female crickets should have a positive response to purring songs. One way to test this idea is to observe whether females move towards a purring song.
She set out to test her hypothesis with phonotaxis experiments in the lab. During phonotaxis experiments, scientists observe how an organism moves with respect to different sounds. In their first experiment, Robin and her colleagues placed a female at the center of an arena and played a purring song through 1 of 4 speakers. The other 3 speakers were silent. To document the female’s willingness to mate, Robin recorded if the female moved toward the purring and which speakers they contacted. If the purring song was not a mating signal, it should not be attractive to the females and she expected them to contact the speakers at random. This would mean that the purring speaker should be contacted 25% of the time (since only 1 of the 4 speakers broadcast purring). If the purring song was a mating signal, she expected female crickets to contact purring speakers more than 25% of the time.
In a second experiment, Robin investigated whether female crickets prefer purring songs as much as typical mating songs. Using the same set-up, she played either a typical or purring song through 1 of 4 speakers. If females moved toward the speaker playing a song before the silent ones, she recorded the search time. Search time was calculated as the time it took the female to contact the broadcasting speaker minus the time at which the crickets started looking for the speaker. To see whether the purring song was evolving as a mating signal, she compared the time it took crickets to find speakers broadcasting the purring song compared to the typcal mating song. She predicted that if females still preferred the typical song more than the new song, that they would have longer search times for purring versus typical songs.
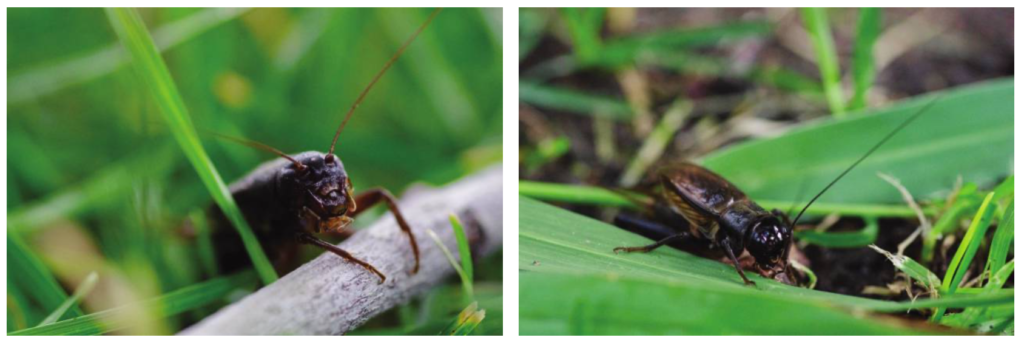
Featured scientist: Robin Tinghitella from The University of Denver.Written by: Gabrielle Welsh
Flesch–Kincaid Reading Grade Level = 9.3
Additional teacher resources related to this Data Nugget include:
- For more information on rapid evolution of cricket song in Hawaii, and to hear sound clips of the purring song, visit Robin’s website!
- The paper published using these data:
- Robin M. Tinghitella, E. Dale Broder, Gabrielle A. Gurule-Small, Claudia J. Hallagan, and Jacob D. Wilson (2018) Purring Crickets: The Evolution of a Novel Sexual Signal. The American Naturalist 192(6): 773-782. Click here for a PDF!